Interview with Dr. Mark Cembrowski
- Jasper Delichte
- 6 days ago
- 10 min read
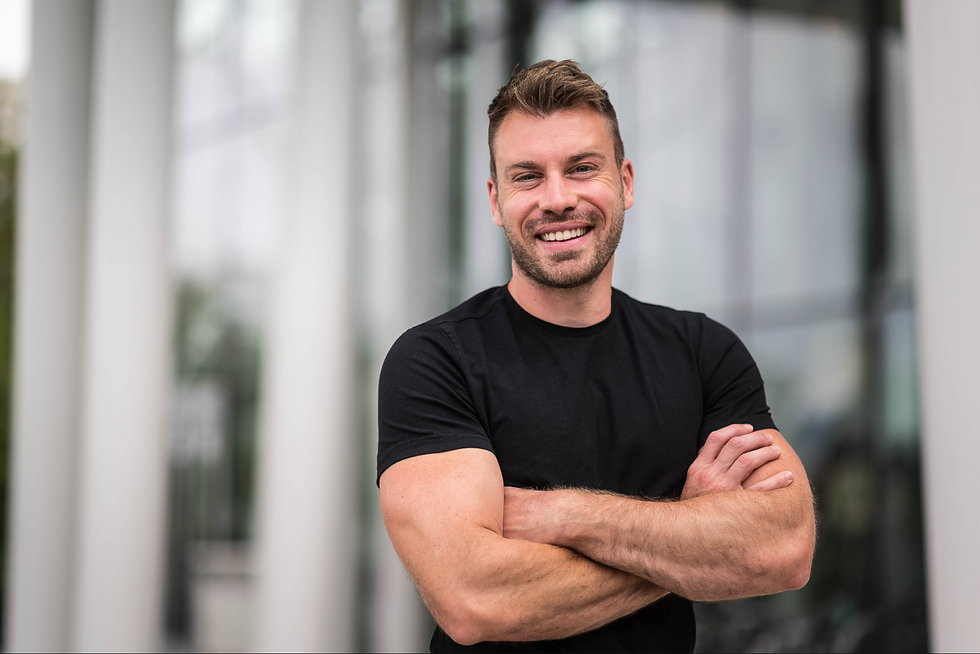
Can you please tell me about yourself and what inspired you to enter research?
My name is Mark Cembrowski and I'm an associate professor at the UBC Faculty of Medicine. As long as I can remember, I've loved learning new things. One of my favorite things to do is feel like I have learned something new everyday and am a little bit smarter than the day before. I have felt like this ever since I was a kid and this is why I have always had a passion for science and what drew me to research. I was once told that the job that you want to have is the thing that you would do if you were retired. And I think if I were to retire tomorrow, I'd keep doing exactly the same thing. So research is my ability to have my passion, just lived out in a professional setting.
That's great. Thank you for sharing. What are some of your research focuses?
Our primary research interests are understanding learning and memory. You remember things, I remember things. But in a lot of ways, we don't actually know what those physical changes are in the brain that give rise to those memories. And so this is one of the key goals that we have for our research group; I think it's one of the foundational goals of neuroscience, and is super important for everything from understanding memory pathologies and Alzheimer's disorder to how memories can change in post-traumatic stress disorder or following traumatic brain injury.
What models, techniques, or systems do you use to study traumatic brain injury?
We have two different models that we use. One is using mice to understand traumatic brain injury, and we have some ways of giving mild traumatic brain injury to mice and using specialized transcriptomics methods to try and understand what happens. We use a lot of data rich techniques. My formal training is as a mathematician, so I want to use techniques where you can get as much data as possible. And so we use those kind of data rich techniques and mouse models. And we're also starting to get involved in using human brain tissue as well from traumatic brain injury to understand directly from the human brain how injury manifest in changes in molecules and cells.
That's very cool that you're able to use human brain tissue as well. Could you please walk us through the CHIMERA model and how transgenic mice are used to study TBI?
So the CHIMERA model is basically a helmet that goes across a rodent, typically it's either a mouse or a rat, and then there's a bonk that's applied to the helmet. But by virtue of this structure, it's a diffuse injury, so it occurs across the extent of the outside of the brain. It can be done in either a mild fashion or with a higher impact. But the general idea behind it is it's a really wonderful model to study what happens in the real world. For example, a lot of sports players sustain many of these mild hits to the head, but over time, this produces traumatic brain injury, and so this is a really good way of studying it in an experimental setting. And another big advantage is that it's reproducible. A lot of models of brain injury that we have right now vary a lot from animal to animal, but this allows a very well controlled way with which we can understand how a single injury manifests in changes across the extent of the brain.
What are transgenic mice?
One of the really neat things about using this in mice is that we can use transgenic approaches that would allow us to do things that we normally couldn't do with human brains. The mouse that we use actually causes changes in fluorescent signaling for neurons that are active during windows of time. So we can see which neurons are active following traumatic brain injury, and use that as a proxy for understanding where activity emerges in the brain, and potentially in the long run, how can we potentially treat injuries in those brain regions?
You use brain-wide imaging. What advantages does it pose compared to traditional, more 2D histological based approaches?
So the traditional 2D approaches, you need someone with really good hands and a lot of patience, because it takes a lot of effort to go through to take a brain and basically cut it section by section, and then to image each one of those sections. With these modern day techniques, you can actually remove a lot of the things that make it relatively opaque so you have a clear, transparent brain, and then you can relabel these fluorescent neurons. And then, in one go, image the entire extent of the brain.
And in terms of behavioral assessments, what are some techniques that you use to evaluate neurological or functional changes following TBI?
Yeah, so this is that work is done in collaboration with Cheryl Wellington and her lab here at UBC. And Mehwish Anwar is the primary postdoctoral researcher that does this kind of work. We have a variety of standard neurological readouts, like the loss of righting reflex is a big one, and just in addition having animals get up and become ambulatory, and so we have some very sort of straightforward behavioral assessments for to look at traumatic brain injury.
How do these studies enhance our understanding of how TBI impacts human brains? What are the translational applications of this particular research?
I often view traumatic brain injury as sort of a needle in the haystack problem where a single injury can lead to brain wide effects; and so we need really powerful, technological ways of zooming in on what brain regions, what cells, and what molecules we think are really important to potentially cause these manifestations of injury. And so from this work, our hope is that we can identify not just brain regions that are affected by this injury, but also the constituent cells and molecules. And by knowing which molecules change and what cells and regions, we can hopefully come up with new targeted therapies. Our ultimate goal is to potentially have pharmacologic interventions where we can prevent some of these changes induced by the injury itself, and hopefully mitigate some of the long term mental or physical ramifications following these injuries.
And what new technologies have helped us to better understand TBI, and how do you expect the field to be shaped over the next five or 10 years?
I'm a little bit biased, but one of my techniques that I think is going to be absolutely transformative is a special technique that's called single-cell spatial transcriptomics. The high level view of this is that it allows you to take really large brain sections and zoom in and identify single molecules, individual cells that are changed by traumatic brain injury. And you can do this over huge fields of view. This is a beautiful way of conquering this needle in a haystack problem, where you can look at broad expanses of the brain and then zoom in on particular regions that seem to have really high degrees of susceptibility. And then be able to potentially, using the knowledge of what those molecules do, be able to engineer new types of treatments, new types of pharmacologic interventions that could be immensely transformative. So, we need techniques that solve this needle in haystack problem and these cool data, rich technologies, I think, will be absolutely critical for both our understanding and eventual treatment of traumatic brain injury.
Why are animal models important for research?
Animal models are absolutely critical for doing two things. Number one is having ways that we can understand brain injuries reproducibly from animal to animal. A big challenge with traumatic brain injuries as they occur in the real world is that they can be immensely variable from one person to the next person, so it can be hard to identify common signatures of these injuries. That's something that we can do with these animal models. The other thing that's really beneficial from using animals in our research is if we identify things that we think may be new druggable targets, new ways that we can intervene and prevent some of these manifestations of injuries, we can test that directly in these models in what's called a pre-clinical setting; and if they do look like they can help with these individual animals' quality of life, that gives us immense confidence to then turn around and hopefully take these therapies into the clinic.
And so shifting gears a bit, how does your research explore memory?
We are fascinated by memory. Our memory makes us who we are, we're nothing without our memories. So our research uses a lot of the same techniques that I just discussed in traumatic brain injury, to try and get at what cells and molecules are critical for aspects of memory. And then our hopeful long term goal of this research program is to understand traumatic memories that can occur following a traumatic brain injury or other types of high intensity stress, and then understand how those are laid down and consolidated in the brain. We're trying to come up with new ways that we can prevent those memories moving from short term to long term memory in the same way that if one goes through something traumatic, like a motor vehicle accident, and first responders come to the scene to treat physical injuries, that may also be a window with which they can treat long term mental ramifications of those injuries as well.
Thank you for sharing. I was reading an article and it was talking about using Tetris following traumatic incidents. Any comments on this research?
I find that study to be incredibly compelling. This goes to the same idea that we're working at here, with the idea of memories having a window of time with which they move from short term to long term memory. That's a process called, consolidation and there's a huge amount of energy in the field to prevent consolidation of traumatic memories, and that Tetris approach is one of those approaches that we believe interferes with that consolidation. The Tetris approach may not be available to all people all the time, and there might also be ways that we can more selectively intervene for the formation or the extension of these long-term fear memories. And so this is what we're going after from understanding what changes in the brain and ultimately, to hopefully come up with new drugs targets where we can do things that are similar to this Tetris approach and potentially complement it and enhance it.
What do you think it is about playing Tetris that disrupts the consolidation process?
Nobody knows. But, we think it's just putting your brain into a state where its normal processes would not occur. Consolidation typically requires the brain to be in an offline state. This can be rest. It can be sleep. So by taking the brain and taking it out of the state where it can undergo consolidation is probably why this has a therapeutic effect; but in reality, we don't know, this just our best guess.
So your team recently discovered a new cell type involved in memory. Could you please elaborate on this discovery?
So one of the brain regions that we've known to be critical for memory is called the hippocampus. The hippocampus is involved in short term memory formation and it's allowance to move into long term memory. The textbook definition of what we call a principal cell type in the hippocampus is the pyramidal cell. These have been studied for decades now. We believe that this cell type has been critical for forming subtypes of new memories. But it's really striking that this one kind of cell type can seemingly do so many different things that are so diverse in learning, memory, and cognition. Our group sort of found that surprising and we thought all right, maybe there's more to it than just this pyramidal cell type and by applying these new types of methods we were able to identify this other excitatory neuron that sits right next to these pyramidal cells. These cells look a little bit different from pyramidal cells, which are called pyramidal cells because they have a triangle shaped cell body. These cells that we found have an oval shaped cell body. So we call them ovoid neurons, and they basically break the rule of everything that we ever thought about pyramidal cells and how they lead to memory. And these ovoid neurons have their own network neural networks that they're embedded in. They communicate very different information about specific forms of memory, and functionally, they can dissociate or bring different forms of memory relative to our classical parental cells. So what this looks like is a new computational layer in the brain involved in very specific types of memory.
That's super cool, thank you for sharing. And so what are your next steps with this research?
We think that the cell type is dysregulated both in epilepsy, as well as Alzheimer's disease, and so we're trying to understand how is it dysregulated. And then are there ways that we can change the activity of these neurons to prevent manifestations of either seizures and epilepsy or a spread of a toxic protein that's called amyloid beta in the context of Alzheimer's disease. If either one of these works out, I think we're in an immensely advantageous spot to come up with new treatments for these sorts of brain disorders.
Thats incredible. How do you think it's implicated in epilepsy?
Yeah, in the sort of healthier, neurotypical brain, these cells are more excitable relative to pyramidal cells. This means that a little bit of current goes a long way in turning these cells on. What we think occurs in epilepsy, is they get turned on a little bit too much that there's some sort of reworking within the network that causes these cells to be overly excitable and actually initiate seizures. And if indeed this is the case, then we can probably come up with ways of targeting this specific cell type to prevent seizure initiation and thereby propagation. And we have some really exciting targets within these cells that there are molecules that already have FDA approved drugs that we can now try and repurpose in the context of epilepsy.
What part of your work do you wish others understood better?
I think I wish people better understood the importance of what we would call foundational or basic neuroscience. This is something that we see a lot in the United States, where a lot of their foundational research is getting curtailed. A lot of strides that we're able to make in a clinic and help individuals that have brain injuries, brain disorders, brain diseases, those are happening now because of foundational research, or curiosity driven research that happened 20, 30, 40, sometimes even 100 years ago. And so I view modern day science and clinical practice as research that we have in sort of clinical aspects right now that funds current day therapies; but research that we're doing that's just curiosity driven, that funds the new therapies of tomorrow. And just doing science for science sake, and figuring out how things work, I think, will be immensely important for how we can understand brain disorders.